More than two millennia of history, including several centuries of industrial development, have told us that energy services are essential to support human development and improve quality of life. More recently, the emergence in policy making of the concept of sustainable development has highlighted the need for an integrated approach to economic development taking into account careful management of natural resources, including our environment, and aiming at passing on to future generation increasing assets.
Research and development, and technological progress, have a major role to play in designing and implementing energy systems that will meet future requirements of society. In this context, the potentials of nuclear energy are very broad. Nuclear energy became a reality very recently and it is only in the second half of the 20th century that nuclear power plants began to be built on a commercial basis. Today, however, the nuclear power industry has reached commercial maturity, accumulated extensive operational experience and established a robust infrastructure. Therefore, nuclear energy offers the opportunities for scientific breakthroughs of a rather young technology, as well as the robust basis of an established industry.
As an introduction to my presentation, I would like to put future technological breakthroughs and potentials of nuclear energy into perspective by reviewing briefly the present contribution of nuclear power to energy supply and the assets that the nuclear sector represents already. Also, I will review the expected characteristics of future energy markets in order to set up the backdrop for nuclear energy systems and investigate how nuclear energy should evolve to match better the requirements of tomorrow’s consumers.

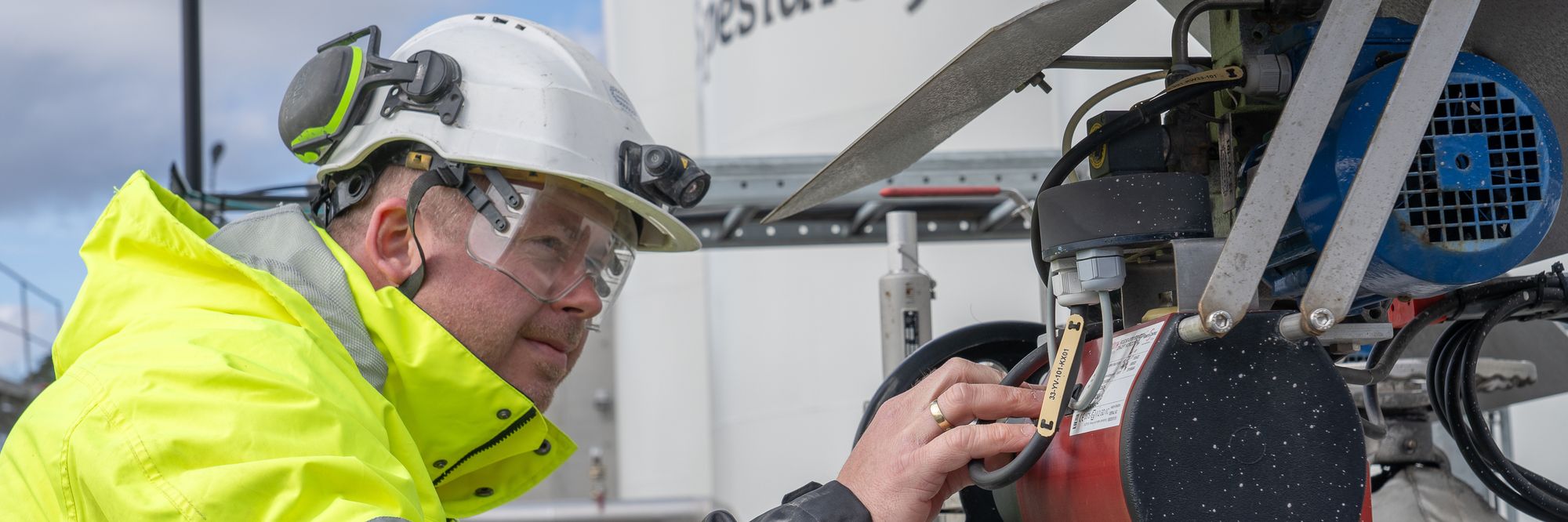
Turning to potentials and breakthroughs, I will not try to be exhaustive since ongoing R&D and development activities in the field of nuclear energy cover a wide range of promising technological options. Rather, I will focus on a few illustrative examples of advanced technologies and innovative concepts that could reach industrial maturity in the coming decades and enhance the contribution of nuclear energy to sustainable development.
In my concluding remarks, I will share with you some findings and thoughts on the role of international co-operation and intergovernmental organisations in facilitating the implementation of R&D programmes that could support a more efficient realisation of technological breakthroughs necessary for making possible the development of nuclear energy systems adapted to the needs of the 21st century.
Today’s assets
In the field of energy supply, changes are slow owing to the long lead-times needed to modify existing systems, e.g., power plants and electricity grids, and to the inertia inherent to sophisticated, capital intensive infrastructures. Therefore, innovations do not penetrate rapidly on energy markets, as demonstrated for example by the trends in renewable source shares in energy supply, and generally lead times from technological breakthroughs to commercial deployment are longer than a decade.
In this context, it is important to note that nuclear power is already a significant component of energy supply worldwide. In more than 30 countries, including 16 OECD countries, nuclear energy is part of the energy mix. Nuclear power is a mature technology that has accumulated around 8 000 reactor years of commercial operation in OECD countries, and nearly 10 000 reactor years worldwide. It provides some 6% of the primary energy consumed in the world and its share in electricity generation exceeds 15%. In OECD countries, some 350 nuclear power plants are connected to the grids and account for nearly 25% of total electricity generation.
The nuclear power plants of current generation have proven to be safe and economic, including in newly deregulated electricity markets of several OECD and other countries. Most of the reactors in operation have not by far reached the end of their technical lifetime and will continue to produce electricity during two or more decades. Even in scenarios that exclude new nuclear power plant construction beyond present committed projects, such as the Reference Case of the IEA (International Energy Agency) World Energy Outlook 2000, the installed nuclear capacity worldwide increases slightly until 2010 and remains more or less stable up to 2020.
The future of nuclear energy will depend upon the records of nuclear power plants in operation in the world today as well as on the success of ongoing nuclear energy R&D programmes. Technical progress has been essential for the enhancement of safety, technical and economic performance of existing nuclear units. Evolutionary developments have achieved improvements in reliability and availability of reactors resulting in electricity generation cost reduction, and incorporate various technical features to meet increasingly stringent safety requirements. Improved performance has been supported by better application of classic technologies as well as the introduction of new technologies with regard to materials and computerised information processing for surveillance and diagnostics.
The nuclear energy industrial and legal infrastructure, R&D equipment and programmes, and human capital in the form of scientific and technical knowledge represent an asset for the continued operation of existing nuclear units, including for extending their lifetime. Moreover, it offers the framework and building blocks for the development of new nuclear energy technologies to be deployed during the 21st century and beyond.
Tomorrow’s market requirements
The analysis of future energy demand characteristics is a prerequisite to relevant choices in the field of nuclear R&D, leading to the development of technologies and concepts adapted to expected market requirements. The types of energy services that society will look for in the 21st century likely will differ from present uses and needs both in quantity and in quality. Population growth, urbanisation, deployment of computers and telecommunication technologies, decentralisation, market liberalisation and increased awareness of environmental issues are among the many factors that will affect energy demand.
The impacts of those factors on characteristics that will be required for future nuclear energy technologies to be successful are varied and somewhat contradictory. The new century will call for energy sources economically competitive in deregulated markets, accepted by the public, benign for human health and the environment as well as sustainable from the view point of natural resource management. Designing nuclear energy systems offering lower cost, enhanced flexibility and higher level of safety than current reactors will raise some challenges. Such achievements will necessitate continued and sustained progress in science and technology.
The evolution towards energy service supply is a major trend in the energy sector. Nuclear fission reactors have demonstrated already their ability to supply electricity and, to a lower extent, heat since a few nuclear units in the world are used for district and/or process heat generation. The energy produced by nuclear reactors may be used also for potable water production, a service for which demand is expected to increase rapidly in many regions. Finally, nuclear energy can play a significant role for large-scale production of hydrogen, if and when hydrogen would become a major secondary energy carrier.
Economic competitiveness remains a cornerstone for industrial and commercial development of new technologies. In the case of nuclear energy, this will imply for new advanced systems significant capital cost reduction as compared with the present generation of reactors. Furthermore, competing successfully in deregulated markets will require enhanced flexibility, that could be obtained for example by modular units better adapted to decentralised electricity supply and uncertainties on demand. Also, thermodynamic efficiency, provided for example by high temperature reactors, can contribute to better economic performance through higher total energy output per unit of fuel.
In terms of health and environmental benignity, nuclear power plants of current generation are very satisfactory, but safety requirements for new nuclear technology are increasingly stringent mainly because of the need to gain public acceptance. The approach adopted by designers to achieve high safety levels generally relies on a combination of passive and active systems taking into account the reliability and economics of both types of systems.
Potentials of nuclear energy technologies for the 21st century
The development of nuclear energy technologies may take several paths in response to the needs and requirements of the 21st century. Advanced fission reactor designs and fuel cycle options are studied by many research teams in different countries along two main lines of programmes: evolutionary approaches and innovative concepts. Both approaches are promising and may lead to more efficient technologies, enhanced safety and better economics. Scientists and engineers are investigating also the possibilities of retrieving energy from nuclear fusion on an industrial scale and fusion systems based upon deuterium fuel could, in the long term, become a practically inexhaustible source of energy.

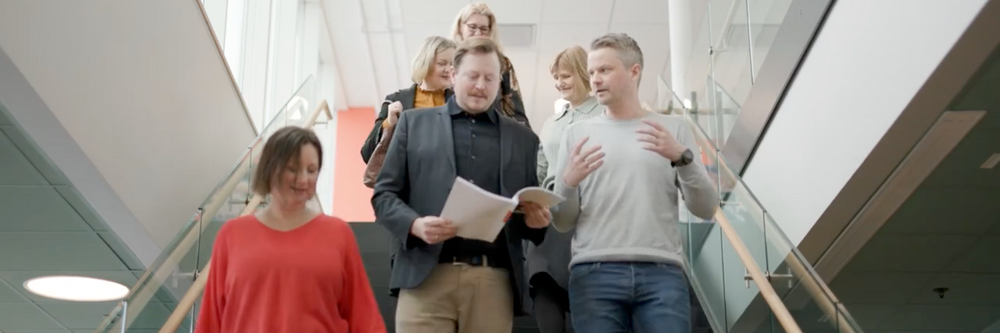
Many governmental organisations, national laboratories, universities and private companies are devoting considerable efforts world-wide to develop advanced nuclear energy technologies. In OECD countries, government expenditures for nuclear R&D, including fission and fusion, have been estimated at nearly 4 billion USD per year by the end of the 20th century.
Although nuclear fusion reactions power the universe, research on harnessing fusion as an industrial energy source is still at an early stage of development and ongoing programmes focus on establishing the feasibility of continuous energy production from a fusion reaction. Thermonuclear fusion energy research is aiming at designing and implementing methods to confine a plasma on earth in a similar way as gravitational forces confine it in stars and the sun. Two very different approaches are pursued at present: magnetic confinement, mainly using toroidal configurations; and inertial confinement, with emphasis on the former that has provided more promising results so far.
The largest international endeavour in the field of thermonuclear fusion R&D is the International Thermonuclear Experimental Reactor project (ITER) that follows the toroidal magnetic confinement approach. The project builds upon the experience acquired with several experimental machines, such as the Joint European Torus (JET) and the American Tokamak Fusion Test Reactor (TFTR). ITER is developing the detailed design concept of a machine that should demonstrate ignition in a large plasma volume and maintain fusion reactions for quasi-steady conditions. The ITER project is carried out jointly by the European Union, Switzerland, Japan and Russia, and recently Canada has expressed interest in being the host country for the demonstration machine.
Regarding fission, a full spectrum of programmes are devoted to different designs and concepts including light and heavy water reactor types, liquid metal cooled fast neutron reactors, high temperature gas cooled reactors, accelerator driven systems, thorium fuel cycles.
One example of integrated approach to research and development of a new generation of nuclear energy systems for the next century is given by the US initiative “Generation IV”. Such an endeavour aims at a comprehensive coverage of issues and topics in order to: identify the future needs and the desired characteristics of new nuclear energy technologies; review the ongoing active R&D efforts in the field; and draw a road map to adapt the basic and applied research programmes to the requirements.
Some of the concepts and designs that may enhance the performance of nuclear energy are very close to commercial development. Evolutionary fission reactor designs, already used in some reactors in operation or under construction, take advantage of scientific and technological progress in various fields such as materials and computer sciences. Further development of new materials more resistant to corrosion is sought in order to reach higher performance, increased reliability and extended lifetime of reactor components. The use of digital computerised systems have helped to improve the performance of existing nuclear power plants. It is expected that technology progress in this field will contribute to enhance nuclear safety, reduce costs, increase operation effectiveness and plant availability, and meet better the needs of plant staff and corporate business strategy.
Research programmes in the field of advanced nuclear systems include many elements that are needed to support the completion of new reactors based upon innovative concepts. The development or enhancement of a large number of enabling technologies is pursued actively as a complement to the establishment of system concepts and designs. Promising enabling technologies range from fundamentally new materials and chemistry control processes to the enhancement of major component performance.
In developing new concepts, some designers devote large efforts to the implementation of passive safety systems based upon natural convection circulation of fluids such as air, lead, molten salt or water for the transfer of decay heat to the environment under postulated accident conditions. The ability to accurately predict the behaviour of these systems will help in validating their safety performance and may bring significant economic savings.
Several innovative designs, including for example advanced pressurised heavy water reactors/CANDU, molten-salt reactors and liquid metal reactors, take advantage of supercritical conditions in the secondary system in order to increase thermodynamic efficiency and reduce costs. The design of reliable primary heat exchangers for operation under supercritical conditions, and the establishment of appropriate chemistry conditions and process control, will require significant development work but is expected to bring important performance enhancement.
Advanced methodologies and procedures to control reactor coolant system chemistry in innovative reactors, in particular those using supercritical conditions, are developed aiming at ensuring longer technical lifetime of components, increase the reliability of the overall system and reduce radiation exposure of operation staff as well as radioactive releases to the environment.
Research on innovative fuel concepts are aimed at ensuring enhanced and reliable performance in the reactors under normal and postulated accident conditions. In the case of high temperature gas cooled reactors, for example, progress is expected in establishing quality control and inspection procedures adapted to current and future safety requirements.
The development of thorium fuels is considered for several innovative reactors and fuel cycle concepts, including high temperature reactors, some liquid metal reactors and the accelerator driven energy amplifier system. The use of thorium broaden significantly the natural resource base for nuclear energy since thorium is estimated to be slightly more abundant on the planet than uranium and has not been extracted on an industrial basis yet.
The ongoing R&D and development programmes on high temperature gas-cooled reactors are illustrative of some prospects for innovative technologies to penetrate on the markets of the new century. Gas cooled reactors offer significant advantages in terms of thermal efficiency and may be used for high temperature process heat applications and for electricity generation as well. Major technological progress in process and component design have led to promising development during recent years. The helium cooled pebble bed modular reactor under development in South Africa is claimed to have the required economic and safety features to meet the expectations of potential investors.
In the field of high temperature reactors, heat exchanger and helium turbine developments are important technological progress milestones that will support the demonstration of the concepts investigated at present. The performance of the heat exchanger incorporated in innovative gas cooled reactor designs could be significantly enhanced and its size reduce by adopting advanced plate type heat exchangers. Various helium turbine developments, partly based upon experience acquired in other industrial sectors using gas turbines, may be considered to enhance reliability and reduce capital costs of gas cooled reactors.
Prospects for nuclear heat applications, especially favourable in the case of high temperature reactors but possible also with other reactors, depend on the adaptation of nuclear technologies to the constraints of heat markets. Nuclear district heating may be considered, as demonstrated by experimental projects in some countries, but will require the development of economically competitive small and medium size reactors, a few hundreds of MWth, with safety characteristics allowing their construction close to urban areas.
Designs that incorporate nuclear power plants on a barge (Russian KLT-60 and Canadian CANBAR 3) offer unique opportunities in term of proliferation resistance and economics. Considerable efforts are required to optimise those designs and the infrastructure to deliver and retrieve the reactor as well as to connect the plants efficiently to local and regional networks, but the economic savings achievable through factory manufacture of components and carrying out decommissioning and waste management in a central location may be very significant.
Several promising liquid metal cooled fast reactor concepts are investigated in different countries. The breeding capability of fast neutron reactors is extremely attractive in terms of natural resource utilisation and management; it may be exploited in the long term to secure energy supply when uranium resources will decrease leading to higher costs and eventually larger environmental impacts (when lower grade ores will have to be extracted).
In the near and medium term, liquid metal cooled fast reactors offer opportunities to manage plutonium and radioactive waste. They may be used for burning actinides and thereby reducing the burden of long-lived radioactive waste for future generation as well as reducing the stock of fissile materials (plutonium) when their core geometry and composition are set up for a conversion ratio lower than one.
The integral fast reactor concept, developed in the United States, has the specific characteristic of a fuel cycle based on metallic fuel and pyrometallurgical processing. Since the fuel is reprocessed and a new fuel is fabricated at the reactor site, the proliferation resistance of the system is very high. The closed cycle on the same site also provides advantages in terms of economics and waste management. The fuel efficiency, capability to achieve high burn-up and passive safety systems of the integral fast reactor are very promising features to match expected market requirements.
Accelerator driven systems (ADS) have raised an increasing interest during the past years and are considered a valuable option for future advanced nuclear fuel cycle options in particular to reduce the inventory of actinides in radioactive waste. Several OECD countries pursue active R&D programmes in this field, aiming in some cases at building a demonstration plant by 2015-2020. In a double strata scenario where the first stratum aims at the production of energy in light water reactors using uranium fuel and plutonium recycling in fast burner reactors, the remaining minor actinide fraction could be burned using accelerator driven systems to reduce the mass and radioactivity of the long-lived radioactive waste by at least a factor of 100.
The few examples given above illustrate the wealth of potentials offered by nuclear energy that could become a reality in the 21st century, provided adequate scientific research is undertaken and timely technological development carried out. The industrial nuclear power plants and fuel cycle facilities that today generate cheap, safe and reliable electricity are based upon scientific research and technical development undertaken as early as the beginning of the 20th century. The future of nuclear energy in the 21st century and beyond depends on research programmes that are undertaken today.
Concluding remarks
At the beginning of the 21st century, in a world that will need increasing quantities of energy and will want to preserve its environment, nuclear energy has large potentials. It can supply a significant share of the services that people need at affordable costs and without jeopardising natural resources and the environment. Realising the potentials of nuclear energy, however, will require sustained R&D efforts covering a wide range of disciplines and technologies.
Furthermore, addressing public concerns about nuclear risks is essential to meet the social objectives of economic development within a sustainable framework. This will require research and development aiming at enhancing nuclear safety and implementing technically sound solutions for the disposal of long-lived radioactive waste. In addition, it will be important to ensure public access to comprehensive information on all the facets of nuclear energy and to create the conditions for a broad participation of all stakeholders in the decision-making process relative to nuclear energy projects at the national and local level.
International co-operation offers unique opportunities to maintain a significant R&D effort momentum while monitoring expenses in an increasingly competitive economic context. One of the key added values of international endeavours is to bring synergy and enhance the efficiency of national programmes. Pooling resources together and carrying out jointly capital or manpower intensive studies not only reduces the cost for each participant country but also offers opportunities for creating more dynamic scientific teams in a multi-cultural environment.
Given its experience in joint projects and its structure adapted to international co-operation, the NEA can play an important role as a catalyst in support of ambitious R&D endeavours for a successful future of nuclear energy. The expertise and management skills available within the NEA secretariat may provide interested Member countries with a robust and flexible framework to efficiently carry out background studies and co-ordinate research projects undertaken by various national teams and laboratories.